Near-Term Quantum Computers
Gain insights into Near-term quantum computers and their profound impact on the field of quantum computing, including technological innovations and practical applications.
Contextualizing Near-term Quantum Computers
As we stand on the brink of a new era in computing, the term "quantum frontier" captures the essence of a transformative journey from classical to quantum computation. Within this domain, Near-term Quantum Computers have emerged as pivotal players. NTQCs represent a category of quantum devices that leverage quantum mechanics for problem-solving while existing within the limitations of current technology. Unlike fully developed quantum computers, which remain largely theoretical and technologically challenging, NTQCs are practical implementations that serve as a bridge to the ultimate goal of quantum supremacy—the point at which quantum computers will outperform classical counterparts in all tasks.
The significance of NTQCs in today's technological landscape cannot be overstated. As industries strive for more efficient algorithms and faster data processing, NTQCs offer a glimpse into the future capabilities of quantum computing. They are particularly crucial now because they provide tangible results and applications, albeit in a limited scope, paving the way for broader acceptance and integration of quantum technologies. With breakthroughs in qubit stability and error correction, NTQCs have reached a threshold where they can address specific challenges in optimization, cryptography, and complex simulations, which are currently intractable for classical computers.
Current trends in quantum computing have seen significant milestones that underscore the importance of NTQCs. For instance, companies like IBM and Google have demonstrated quantum devices that achieve tasks beyond the reach of traditional supercomputers, albeit still confined to narrow domains. Google's Sycamore processor, for example, achieved quantum supremacy by performing a complex computation in just minutes that would have taken the fastest classical computer thousands of years. These developments highlight the potential of NTQCs in shaping future computational landscapes, with predictions indicating that the market for quantum computing could reach $65 billion by 2030. As we continue to explore the frontier of quantum technology, NTQCs stand as a testament to human ingenuity, promising to unlock new possibilities in science, industry, and beyond.
All You Need for Project Management is in Meegle
Conceptual overview
Decoding the Theories Behind NTQCs
Understanding the theoretical foundations of Near-term Quantum Computers involves delving into the complex yet fascinating world of quantum mechanics. At the heart of quantum computing lies the concept of the qubit, the quantum analog of the classical bit. Unlike bits, which can be either 0 or 1, qubits can exist in a state of superposition, meaning they can represent both 0 and 1 simultaneously. This feature allows quantum computers to process a vast amount of information in parallel, offering a significant advantage over classical systems in specific computational tasks.
Quantum mechanics, the fundamental theory underlying quantum computing, introduces several principles that make NTQCs viable. Superposition is one such principle, enabling qubits to perform multiple calculations at once. Another key principle is entanglement, a phenomenon where qubits become interconnected such that the state of one qubit immediately influences the state of another, regardless of distance. This property is leveraged in quantum computing to create correlations between qubits, enhancing computational power and efficiency. Quantum gates, the building blocks of quantum circuits, manipulate qubits using these principles, performing operations that are exponentially faster than classical logic gates.
While NTQCs share these foundational concepts with fully developed quantum computers, they differ in scope and capability. NTQCs are designed to tackle specific problems that offer near-term commercial and scientific benefits, such as optimization and modeling complex systems, whereas fully developed quantum computers aim for broader, more generalized problem-solving applications. This distinction is crucial as it positions NTQCs as practical tools in the current technological landscape, capable of providing immediate value while paving the way for the eventual realization of universal quantum computers.
Core Principles and Mechanics
To fully grasp the workings of Near-term Quantum Computers, it is essential to explore their core principles and operational mechanics. NTQCs function by exploiting quantum phenomena such as superposition, entanglement, and quantum interference, allowing them to execute complex computations with unprecedented speed and accuracy. These principles are implemented through quantum circuits, where qubits are manipulated using quantum gates to perform specific algorithms.
Superposition, as a cornerstone of quantum mechanics, enables NTQCs to evaluate multiple possibilities simultaneously. For example, in solving optimization problems, a quantum computer can explore various configurations at once, rapidly converging on the optimal solution. Entanglement further amplifies computational power by linking qubits in ways that classical systems cannot replicate. This interconnectedness allows for faster data transmission and processing, making NTQCs ideal for tasks involving large datasets and complex interdependencies.
Quantum gates play a crucial role in NTQC operations, functioning as the quantum equivalent of classical logic gates. These gates manipulate qubits to perform specific operations, such as rotations or flips, enabling the execution of quantum algorithms. Unlike classical gates, which operate in binary states, quantum gates leverage the probabilistic nature of qubits, allowing for more nuanced and efficient computations.
A notable aspect of NTQCs is their reliance on hybrid quantum-classical models. These models integrate quantum and classical computing resources to optimize performance and overcome current technological limitations. By combining the strengths of both paradigms, hybrid models enable NTQCs to tackle complex problems that remain challenging for either approach alone. This synergy is particularly evident in applications like machine learning and data analysis, where quantum algorithms enhance classical techniques to deliver superior results. As we continue to explore the potential of NTQCs, understanding these core principles and mechanics is vital, providing a foundation for future advancements in quantum computing.
Click here to read our expertly curated top picks!
Tools and technologies driving ntqcs
Technological Infrastructure
The technological infrastructure supporting Near-term Quantum Computers is a blend of cutting-edge hardware and sophisticated software systems. At the core of this infrastructure are quantum processors, specialized chips designed to manipulate qubits. These processors are housed within cryogenic systems that maintain the ultra-cold temperatures necessary to preserve qubit coherence and minimize decoherence, a major challenge in quantum computing. Companies like D-Wave, Rigetti, and IBM are at the forefront of developing quantum processors, each employing different technologies such as superconducting circuits and trapped ions to harness quantum phenomena effectively.
Cryogenic systems play a critical role in NTQCs by providing the extreme cooling needed to stabilize qubits. Quantum systems operate near absolute zero to reduce thermal noise and enhance the coherence time of qubits, which is essential for maintaining quantum states long enough to perform computations. This requirement poses significant engineering challenges, but advancements in cryogenic technology are enabling more stable and scalable quantum computing environments.
On the software side, quantum algorithms and programming languages are vital components of NTQC technology. Quantum algorithms, such as Shor's algorithm for factoring large numbers and Grover's algorithm for searching unsorted databases, demonstrate the computational advantages of quantum systems. These algorithms exploit the principles of quantum mechanics to solve problems more efficiently than classical counterparts. Quantum programming languages, like Qiskit, Cirq, and Q#, provide developers with the tools to create and optimize quantum algorithms, facilitating the integration of quantum computing into existing workflows.
Several companies and institutions are spearheading NTQC development, each contributing unique innovations and expertise. Google, for example, has made significant strides with its Sycamore processor, which achieved a landmark in quantum supremacy. IBM's Q System One is another example, offering a commercial quantum computing platform that provides cloud-based access to quantum processors. These developments highlight the collaborative efforts across the quantum computing industry, driving advancements in NTQC technology and paving the way for broader adoption in various sectors.
Innovations and Breakthroughs
The field of Near-term Quantum Computers is experiencing a surge of innovations and breakthroughs that are accelerating its development and potential applications. One of the most significant advancements is in quantum error correction, a crucial technique for mitigating errors that arise from qubit instability and environmental interference. Researchers are developing sophisticated error correction codes, such as surface codes and topological codes, which enhance the reliability and accuracy of quantum computations. These innovations are vital for the practical implementation of NTQCs, as they address the inherent challenges of maintaining quantum coherence over extended periods.
Another breakthrough propelling NTQCs forward is noise reduction technology. Quantum systems are highly sensitive to external disturbances, which can degrade qubit performance and lead to errors. Innovations in noise reduction techniques, such as improved shielding and advanced error mitigation algorithms, are enhancing qubit stability and performance. These advancements are enabling NTQCs to perform more complex computations with higher fidelity, bringing them closer to realizing their full potential.
Collaborations and partnerships are also playing a pivotal role in advancing NTQC technology. Leading technology companies, academic institutions, and government agencies are joining forces to accelerate research and development in quantum computing. Initiatives like the Quantum Computing Initiative by the U.S. Department of Energy and the European Union's Quantum Flagship program are fostering collaboration across borders and disciplines, driving innovation and setting the stage for the future of quantum computing. These partnerships are facilitating knowledge sharing, resource pooling, and the establishment of industry standards, all of which are essential for the growth and success of NTQCs.
In summary, the innovations and breakthroughs in NTQC technology are laying the groundwork for a quantum revolution. By addressing challenges such as error correction, noise reduction, and scalability, these advancements are making NTQCs more viable and effective, paving the way for their integration into various industries and applications. As collaboration and innovation continue to flourish, the future of NTQCs looks promising, with the potential to redefine the limits of computation and unlock new possibilities for scientific discovery and technological advancement.
Applications and use cases
Industry Applications
Near-term Quantum Computers are poised to revolutionize industries by providing solutions to complex problems that classical computers struggle to address. One of the most prominent applications is in cryptography, where NTQCs offer significant advantages in both encryption and decryption processes. Quantum algorithms, such as Shor's algorithm, can efficiently factor large numbers, posing a threat to traditional cryptographic systems like RSA. However, NTQCs also provide opportunities for developing quantum-safe encryption methods, ensuring secure communications in the quantum era.
In the finance sector, NTQCs are transforming algorithm optimization and risk analysis. Quantum computers can rapidly analyze vast datasets, identifying patterns and insights that were previously inaccessible. This capability enhances decision-making processes, enabling financial institutions to optimize portfolios, manage risk more effectively, and improve market predictions. For instance, NTQCs can solve complex optimization problems in seconds, providing a competitive edge in high-frequency trading and investment strategies.
The healthcare industry stands to benefit significantly from NTQCs in drug discovery and personalized medicine. Quantum simulations can model complex molecular interactions with high precision, accelerating the drug discovery process and reducing costs. NTQCs enable researchers to explore vast chemical spaces, identifying promising drug candidates and optimizing molecular structures for desired properties. This capability has the potential to revolutionize the development of new therapies, particularly for complex diseases such as cancer and neurodegenerative disorders.
Logistics and supply chain management are also being transformed by NTQCs. Quantum algorithms can optimize routing and scheduling, improving efficiency and reducing costs in transportation and distribution networks. By analyzing large datasets in real time, NTQCs enable companies to adapt to changing conditions and optimize resource allocation, leading to more sustainable and efficient operations.
Real-world Examples and Case Studies
Several real-world examples illustrate the transformative potential of Near-term Quantum Computers in various industries. Google's quantum computing team made headlines with their demonstration of quantum supremacy using the Sycamore processor. This achievement involved a complex computation that would have taken the fastest classical supercomputer thousands of years, showcasing the power of NTQCs in solving specific tasks with unprecedented speed and efficiency. This milestone highlights the potential of NTQCs to address problems beyond the reach of classical systems, paving the way for new applications and innovations.
IBM is another key player in the NTQC landscape, offering a range of quantum computing solutions through its IBM Quantum Experience platform. This cloud-based service provides users with access to real quantum processors, enabling researchers and developers to experiment with quantum algorithms and applications. IBM's collaboration with companies across various sectors, including finance, healthcare, and logistics, demonstrates the versatility and potential of NTQCs in solving industry-specific challenges.
In the logistics sector, Volkswagen has partnered with D-Wave to explore quantum computing applications in traffic optimization. By leveraging NTQCs, Volkswagen aims to improve traffic flow and reduce congestion in urban areas, leading to more efficient and sustainable transportation networks. This collaboration highlights the potential of NTQCs to enhance operational efficiency and address complex logistical challenges.
Government and academic research initiatives are also contributing to the advancement of NTQCs. The U.S. Department of Energy's Quantum Computing Initiative and the European Union's Quantum Flagship program are fostering collaboration and innovation across the quantum computing landscape. These initiatives support research and development efforts, promoting knowledge exchange and resource sharing to accelerate the integration of NTQCs into various industries.
In summary, real-world examples and case studies demonstrate the transformative potential of NTQCs in addressing complex challenges across multiple sectors. By harnessing the power of quantum mechanics, NTQCs offer solutions that were previously unattainable, paving the way for new applications and innovations. As collaboration and research continue to drive advancements in NTQC technology, the impact of quantum computing on industries and society is set to expand, unlocking new possibilities and opportunities for growth and development.
Click here to read our expertly curated top picks!
Overcoming technical obstacles
Major Challenges in NTQC Implementation
Despite the promising potential of Near-term Quantum Computers, several technical challenges must be addressed to realize their full capabilities. One of the most significant obstacles is the high error rates and decoherence associated with qubits. Qubits are highly sensitive to environmental disturbances, leading to errors in quantum computations. Decoherence, the loss of quantum coherence, poses a major challenge in maintaining qubit stability and performance over time. Addressing these issues is crucial for ensuring the reliability and accuracy of NTQC operations.
Scalability is another critical challenge in NTQC implementation. Building scalable quantum systems requires the integration of a large number of qubits while maintaining coherence and minimizing errors. Current quantum processors are limited in size and capability, with most systems comprising only a few dozen qubits. Achieving scalability involves overcoming significant engineering and technological hurdles, including developing efficient qubit control mechanisms and error correction techniques.
The complexity of quantum circuitry is also a major challenge in NTQC development. Designing and optimizing quantum circuits requires a deep understanding of quantum mechanics and the interactions between qubits. This complexity poses significant challenges in developing efficient quantum algorithms and optimizing qubit interactions for specific applications. Furthermore, the integration of quantum and classical components in hybrid models adds additional layers of complexity, requiring sophisticated software and hardware solutions to ensure seamless operation.
Solutions and Research Directions
To overcome the technical challenges facing Near-term Quantum Computers, researchers are exploring a range of solutions and research directions. Advanced materials and qubit technologies are being developed to enhance qubit coherence and stability. For example, topological qubits, which are less susceptible to environmental disturbances, offer promising potential for improving qubit performance. Researchers are also exploring alternative qubit technologies, such as trapped ions and photonic qubits, to enhance scalability and reduce error rates.
Quantum error correction codes are another key area of research aimed at improving NTQC reliability. These codes, such as surface codes and topological codes, are designed to detect and correct errors in quantum computations, enhancing qubit performance and reducing the impact of decoherence. Ongoing research is focused on developing more efficient error correction techniques that can be implemented in practical quantum systems, paving the way for more reliable and scalable NTQCs.
Artificial intelligence (AI) and machine learning are playing an increasingly important role in addressing NTQC challenges. AI algorithms are being leveraged to optimize quantum circuits, improve qubit control, and enhance error correction techniques. Machine learning models are also being used to analyze large datasets and identify patterns in quantum computations, enabling more efficient and effective quantum algorithms.
Collaborative research initiatives and partnerships are also driving advancements in NTQC technology. Government, academic, and industry collaborations are fostering knowledge sharing and resource pooling, accelerating research and development efforts. These partnerships are facilitating the exchange of ideas and expertise, promoting innovation and the development of new solutions to overcome technical challenges.
In summary, overcoming the technical obstacles facing NTQCs requires a multifaceted approach involving advanced materials, error correction techniques, AI integration, and collaborative research efforts. By addressing these challenges, researchers and developers are paving the way for more reliable, scalable, and effective NTQCs, unlocking new possibilities and applications in quantum computing.
Future directions: the quantum horizon
Predicting Developments in Quantum Computing
The future of quantum computing is poised to be transformative, with Near-term Quantum Computers playing a pivotal role in driving advancements and shaping the quantum landscape. As we look ahead, several key developments and potential breakthroughs are on the horizon. One of the most anticipated advancements is the achievement of quantum supremacy, where NTQCs outperform classical computers in solving specific tasks across a broader range of applications. While initial demonstrations of quantum supremacy have been achieved in controlled experiments, the next step involves expanding this capability to more practical and real-world problems.
The feasibility of quantum supremacy with NTQCs is closely linked to improvements in qubit technologies, error correction, and scalability. Researchers are actively exploring ways to enhance qubit coherence and reduce error rates, which are critical for achieving reliable and scalable quantum systems. As these advancements continue to unfold, NTQCs are expected to tackle increasingly complex problems in optimization, cryptography, and machine learning, offering solutions that were previously unattainable.
Emerging trends in quantum computing also point to the convergence of quantum technologies with other cutting-edge fields, such as artificial intelligence (AI) and the Internet of Things (IoT). The integration of NTQCs with AI has the potential to revolutionize data analysis and decision-making processes, enabling more efficient and accurate predictions and optimizations. Similarly, the fusion of quantum computing with IoT could lead to the development of smarter and more connected devices, enhancing the capabilities of IoT networks and applications.
Potential Breakthroughs and Implications
The potential breakthroughs in Near-term Quantum Computers have profound implications for various industries and sectors. One of the most significant impacts is expected in the field of cryptography, where NTQCs could render traditional encryption methods obsolete. The development of quantum-safe encryption techniques and protocols will be crucial for maintaining data security and privacy in the quantum era. As NTQCs become more widely adopted, industries will need to adapt their security measures to protect sensitive information and communications.
The convergence of NTQCs with other technologies, such as AI and IoT, is expected to drive innovation and growth across multiple sectors. In healthcare, quantum computing could accelerate drug discovery and personalized medicine, leading to more effective treatments and therapies for complex diseases. In finance, NTQCs could enhance risk analysis and portfolio optimization, enabling more informed investment decisions and strategies. The logistics and supply chain sectors could benefit from improved optimization and resource allocation, leading to more efficient and sustainable operations.
As NTQCs continue to advance, their role in achieving sustainable technological growth becomes increasingly important. By addressing complex computational challenges and unlocking new possibilities, NTQCs have the potential to drive innovation and growth across various industries, contributing to a more sustainable and connected future. The continued development and integration of NTQCs into existing technological landscapes will be crucial for realizing their full potential and impact.
In summary, the future of quantum computing holds immense promise, with NTQCs at the forefront of driving advancements and shaping the quantum horizon. As researchers and developers continue to explore new solutions and applications, the potential breakthroughs and implications of NTQCs will have far-reaching effects on industries and society, paving the way for a new era of technological innovation and growth.
Click here to read our expertly curated top picks!
Ethical and societal impact
Broader Consequences of NTQCs
As Near-term Quantum Computers become more integrated into various industries and sectors, their broader societal and ethical implications must be carefully considered. One of the primary concerns is data privacy and security. Quantum computing has the potential to break traditional encryption methods, posing significant risks to data protection and privacy. As NTQCs become more prevalent, industries and governments will need to develop quantum-safe encryption protocols to safeguard sensitive information and communications. Ensuring data security in the quantum era is critical for maintaining trust and confidence in digital systems and services.
The legal and governance challenges posed by NTQCs are also significant. As quantum computing technology evolves, existing legal frameworks and regulations may need to be updated to address new ethical and societal concerns. Policymakers will need to consider the implications of quantum computing on intellectual property rights, data protection, and cybersecurity. Additionally, the development of international standards and agreements will be crucial for promoting responsible and ethical use of quantum technology, ensuring that its benefits are accessible to all while minimizing potential risks and harms.
The socio-economic impacts of NTQCs are another important consideration. As quantum computing technology advances, it has the potential to create new job opportunities and drive economic growth. However, it may also contribute to digital divides and workforce disparities. Ensuring equitable access to quantum technology and education will be essential for promoting inclusivity and diversity in the quantum workforce. As industries and governments adapt to the quantum era, they will need to address potential inequalities and ensure that the benefits of quantum computing are shared broadly across society.
In summary, the broader consequences of NTQCs extend beyond technological advancements to encompass ethical, legal, and socio-economic considerations. As quantum computing technology continues to develop, it will be essential to address these challenges and promote responsible and ethical use of NTQCs. By fostering collaboration and dialogue among stakeholders, we can ensure that quantum computing contributes to a more secure, inclusive, and equitable future.
Step-by-step guide to understanding ntqcs
A Practical Approach to Grasping NTQCs
Step 1: Familiarize with Quantum Basics
Understanding the fundamentals of quantum computing begins with familiarizing yourself with key concepts and terminology. Concepts such as qubits, superposition, entanglement, and quantum gates are the building blocks of quantum computing. By grasping these basic principles, you can better understand how NTQCs operate and their potential applications. Resources such as introductory textbooks, online courses, and educational videos can provide valuable insights into the world of quantum mechanics and computing.
Step 2: Explore Quantum Hardware
The next step in understanding NTQCs involves exploring the components and technologies that make up quantum hardware. Quantum processors, cryogenic systems, and qubit technologies are essential elements of NTQCs. By learning about the different types of qubits and the technologies used to manipulate them, you can gain a deeper appreciation of the engineering challenges and innovations in quantum computing. Visiting laboratories or attending conferences and workshops can provide hands-on experience and exposure to the latest advancements in quantum hardware.
Step 3: Study Quantum Algorithms
Quantum algorithms are at the heart of quantum computing's power and potential. By studying key quantum algorithms, such as Shor's algorithm and Grover's algorithm, you can understand how quantum computers solve complex problems more efficiently than classical systems. Resources such as academic papers, online tutorials, and quantum programming platforms can help you explore the logic and functionality of these algorithms. Experimenting with quantum programming languages like Qiskit and Cirq can also provide practical experience in developing and testing quantum algorithms.
Step 4: Engage with NTQC Applications
Exploring real-world use cases and applications of NTQCs is a vital step in understanding their potential impact. Industries such as cryptography, finance, healthcare, and logistics are already leveraging NTQCs to address specific challenges and optimize operations. By examining case studies and industry reports, you can gain insights into how NTQCs are being applied and the benefits they offer. Engaging with experts and stakeholders in these fields can provide valuable perspectives on the current and future applications of NTQCs.
Step 5: Stay Informed on Latest Developments
The field of quantum computing is rapidly evolving, with new breakthroughs and innovations emerging regularly. Staying informed on the latest research, trends, and developments is essential for understanding the future trajectory of NTQCs. Following academic journals, attending conferences, and participating in online forums and communities can help you stay updated on cutting-edge research and advancements. Engaging with thought leaders and experts in the quantum computing field can also provide valuable insights and inspiration for your own exploration and understanding of NTQCs.
Click here to read our expertly curated top picks!
Do's and don'ts in navigating ntqcs
Do's | Don'ts |
---|---|
Stay updated with the latest research. | Underestimate the complexity of NTQCs. |
Engage in cross-disciplinary learning. | Ignore ethical and societal implications. |
Collaborate with experts in the field. | Focus solely on technical aspects. |
Explore NTQC applications in your industry. | Overlook potential risks associated with NTQCs. |
Click here to read our expertly curated top picks!
Summarizing insights on ntqcs
The Transformative Potential of NTQCs
In exploring the potential of Near-term Quantum Computers, we have uncovered a realm of possibilities that stand to transform industries and redefine the limits of computation. NTQCs, with their ability to harness the principles of quantum mechanics, offer solutions to complex problems that classical computers struggle to address. From cryptography and finance to healthcare and logistics, the applications of NTQCs are vast and varied, promising to enhance efficiency, security, and innovation across multiple sectors.
The ongoing research and development efforts in NTQC technology are crucial for overcoming the technical challenges that currently hinder their widespread adoption. By addressing issues such as error rates, scalability, and the complexity of quantum circuitry, researchers are paving the way for more reliable and effective NTQCs. Collaborative initiatives and partnerships continue to drive advancements, fostering innovation and knowledge sharing in the quantum computing community.
As we look to the future, the potential breakthroughs and implications of NTQCs are immense. The convergence of NTQCs with other cutting-edge technologies, such as artificial intelligence and the Internet of Things, opens new avenues for growth and innovation. By embracing the challenges and opportunities presented by NTQCs, industries and society can unlock a new era of technological advancement and sustainable growth.
In conclusion, the transformative potential of NTQCs is a testament to the ingenuity and determination of researchers and developers in the field of quantum computing. By continuing to explore new solutions and applications, NTQCs have the power to reshape the technological landscape and drive progress in the years to come. As we navigate the quantum frontier, the promise of NTQCs remains a beacon of hope and inspiration for a brighter and more connected future.
All You Need for Project Management is in Meegle
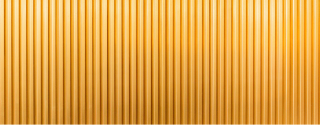
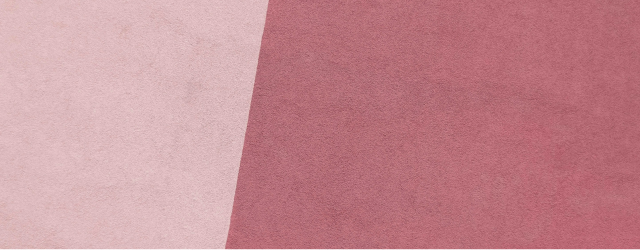
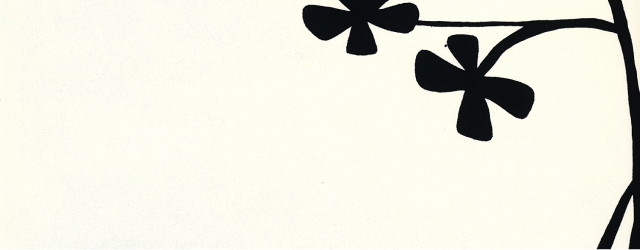
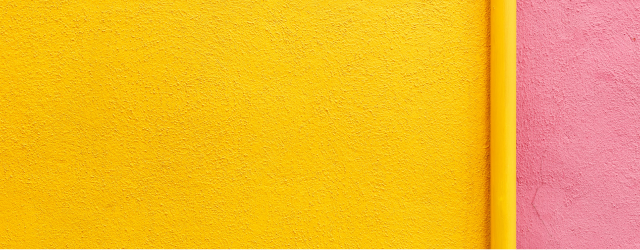
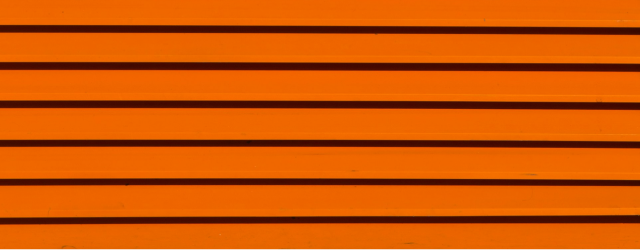
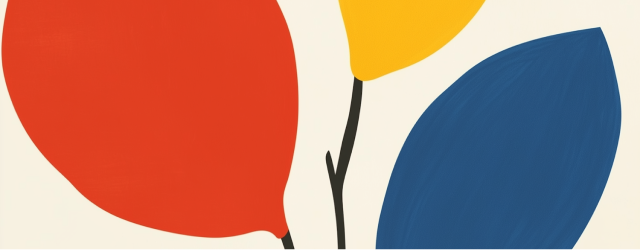
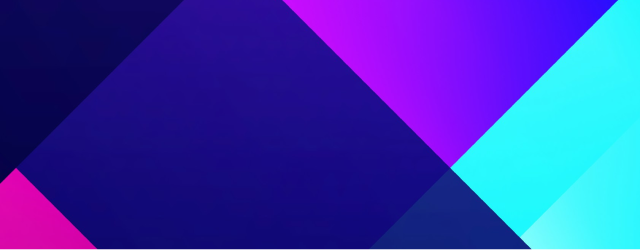
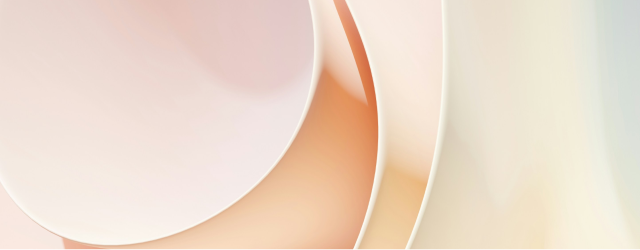