Scalable Quantum Computers
Gain insights into Scalable quantum computers and their profound impact on the field of quantum computing, including technological innovations and practical applications.
Contextualizing Scalable Quantum Computers in Modern Technology
To appreciate the revolutionary potential of scalable quantum computers, it is essential to understand what distinguishes them from classical computers. Classical computers rely on bits, representing either a 0 or a 1, to perform operations. In contrast, quantum computers use qubits, which can represent both 0 and 1 simultaneously thanks to the principle of superposition. This allows quantum computers to process a vast number of possibilities at once, offering exponential speedup for certain computations. However, achieving this potential hinges on scalability, or the ability to efficiently increase the number of qubits used in computation.
The concept of scalability in quantum computing is pivotal. It refers to the capability to build systems that can handle a significant number of qubits while maintaining their quantum state, which is notoriously fragile. This scalability is what will allow quantum computers to tackle complex problems that are currently beyond the reach of even the most advanced classical supercomputers. As industries increasingly require solutions to big data and complex algorithmic challenges, scalable quantum computers are poised to become indispensable tools.
In modern technology, the relevance of scalable quantum computers cannot be overstated. For instance, in logistics, optimizing supply chains involves solving a multitude of variables that classical algorithms struggle with. Scalable quantum systems can address these challenges by processing a large number of variables simultaneously. Similarly, in material science, the development of new materials with specific properties is a combinatorial problem that scalable quantum computers can solve more efficiently by simulating atomic interactions. Thus, the scalability of quantum computers is not merely an academic goal but a practical necessity for their integration into industrial applications. By understanding and overcoming the challenges of scalability, the full potential of quantum computing can be realized, heralding a new era of technological advancement.
All You Need for Project Management is in Meegle
Conceptual overview of scalable quantum computers
Decoding the Complex Theories Behind Quantum Scalability
At the heart of quantum computing are the unique principles of quantum mechanics—superposition and entanglement. These principles not only differentiate quantum computers from classical ones but also underpin their potential for scalability. Superposition allows qubits to exist in multiple states simultaneously, drastically increasing computational power. Entanglement further enhances this by linking qubits in such a way that the state of one instantly influences the state of another, no matter the distance between them. This interconnectedness is crucial for scaling up quantum systems, as it enables complex operations across multiple qubits without the need for physical connections.
The role of qubits in achieving scalability cannot be overstated. Qubits are the fundamental building blocks of quantum computers, analogous to bits in classical computing but with exponentially greater power due to their quantum properties. As more qubits are added, the computational capacity of a quantum system grows exponentially. However, maintaining a large number of qubits in a coherent state, where they can effectively interact and perform calculations, is a significant challenge. This is due to decoherence, a phenomenon where qubits lose their quantum properties due to environmental interactions.
Scalability, therefore, involves not only increasing the number of qubits but also ensuring their stability and accuracy over prolonged periods. Researchers are actively exploring various technologies to achieve this, such as superconducting qubits, which use circuits cooled to near absolute zero to reduce noise and error rates. Moreover, advancements in quantum error correction, which involves using additional qubits to identify and correct errors in computation, are critical for scalable systems. By leveraging these principles and technologies, scalable quantum computers can process vast amounts of information simultaneously, unlocking solutions to complex problems that classical computers cannot handle efficiently.
Core Principles and Mechanics Empowering Scalable Quantum Computers
The architecture of scalable quantum computers is built upon several core principles and mechanics that enable their operation and potential for scalability. Central to this architecture are quantum gates and circuits, which perform operations on qubits analogous to logic gates in classical computers but with the added complexity of quantum states. Quantum algorithms, such as Shor's algorithm for factoring large numbers or Grover's algorithm for searching unsorted databases, exploit these quantum operations to achieve speedups over classical algorithms.
One of the significant challenges in scaling quantum computers is managing coherence time, the duration for which qubits maintain their quantum state. Longer coherence times allow for more complex computations but are difficult to achieve due to the sensitivity of qubits to environmental noise. To enhance coherence, researchers use various techniques such as isolating qubits from external fields and employing error correction protocols. Error rates are another critical factor; even a single error in quantum computation can lead to incorrect results. Therefore, fault tolerance, the ability to continue functioning correctly despite errors, is essential for scalable quantum systems.
Breakthroughs in quantum error correction have been pivotal in addressing these challenges. These techniques involve using additional qubits to detect and correct errors, allowing computations to proceed accurately. For example, the surface code is a leading error correction method that arranges qubits in a two-dimensional lattice, enabling robust error detection and correction. These advancements are crucial for making quantum computers scalable, as they provide the reliability needed to perform complex, long-duration computations.
By integrating these core principles and mechanics, scalable quantum computers can potentially revolutionize various fields. As research progresses, overcoming the challenges of coherence, error rates, and fault tolerance will be key to unlocking the full potential of quantum computing, paving the way for unprecedented computational capabilities.
Click here to read our expertly curated top picks!
Tools and technologies driving scalable quantum computers
Technological Infrastructure Supporting Quantum Scalability
The technological infrastructure that supports quantum scalability is a tapestry of advanced hardware and software innovations designed to overcome the limitations of current quantum systems. At the forefront are technologies like ion traps, superconducting qubits, and photonic systems, each contributing uniquely to the development of scalable quantum computers. Ion traps use electrically charged atoms confined and manipulated using electromagnetic fields to maintain qubit states with high precision. Superconducting qubits, on the other hand, leverage superconducting circuits at ultra-low temperatures to minimize noise and maintain coherence. Photonic systems utilize photons as qubits, offering the advantage of operating at room temperature and facilitating easier integration with existing optical technologies.
Moreover, hybrid computing approaches are gaining attention for their potential to seamlessly integrate classical and quantum systems, enhancing scalability. These systems leverage the strengths of both paradigms, using classical processors to manage tasks like error correction and state preparation, while quantum processors tackle complex computations. This synergy not only boosts the performance of quantum systems but also provides a practical pathway for integrating quantum computing into existing computational frameworks.
Software advancements are equally crucial in supporting quantum scalability. Quantum algorithms are being refined to optimize performance on scalable systems, while quantum programming languages and platforms are being developed to facilitate the implementation of these algorithms. For example, languages like Qiskit and Cirq offer tools and libraries for developing and testing quantum applications, making it easier for researchers and developers to engage with quantum computing.
By advancing these hardware and software technologies, the foundation for scalable quantum computers is being laid. These innovations are crucial for overcoming the inherent challenges of quantum computing, such as qubit coherence and error rates, and for realizing the full potential of quantum systems in solving real-world problems.
Innovations and Breakthroughs in Quantum Scalability
Recent innovations and breakthroughs are accelerating the journey toward scalable quantum computers, bringing what was once a theoretical concept closer to practical reality. One of the most promising advancements is quantum annealing, a specialized form of quantum computing that excels at solving optimization problems. Quantum annealers, such as those developed by D-Wave Systems, use a process akin to simulated annealing to find the optimal configuration of variables, offering a scalable approach to certain types of computations.
Another groundbreaking development is topological qubits, which hold promise for improving scalability by enhancing error resistance. Unlike traditional qubits, topological qubits store information in the global properties of the quantum state, making them inherently more stable against local disturbances. This stability could lead to more robust and scalable quantum systems, as fewer resources would be needed for error correction.
Collaborations between academic institutions, tech giants, and startups are also playing a critical role in advancing quantum scalability research. For instance, companies like IBM, Google, and Microsoft are investing heavily in quantum research, developing new hardware and software solutions to tackle the challenges of scalability. Academic institutions are contributing through cutting-edge research and the development of innovative technologies, while startups like Rigetti Computing and IonQ are pushing the boundaries of what is possible with scalable quantum systems.
These collaborations are fostering a vibrant ecosystem of innovation, driving rapid advancements in the field of quantum computing. As these breakthroughs continue to unfold, the path toward scalable quantum computers becomes clearer, promising to unlock new possibilities across a wide range of industries and applications.
Applications and use cases of scalable quantum computers
Quantum computing applications across industries
Quantum computing applications across industries
Scalable quantum computers have the potential to revolutionize industries by solving complex problems more efficiently than classical systems. In cryptography, quantum computers can enhance encryption methods, providing unprecedented security for data transmission. Quantum key distribution (QKD) is a notable application, offering theoretically unbreakable encryption by leveraging the principles of quantum mechanics. This ensures secure communication channels, safeguarding sensitive information in sectors such as finance and defense.
In the financial industry, scalable quantum computers can optimize portfolio management and risk assessment by processing vast datasets and identifying patterns that are invisible to classical algorithms. Quantum algorithms, like the variational quantum eigensolver, can model complex financial systems to predict market trends and optimize investment strategies, offering a competitive edge to firms that adopt this technology.
The healthcare sector stands to benefit significantly from scalable quantum computing. In drug discovery, quantum computers can simulate molecular interactions at a quantum level, providing insights into the behavior of complex biological systems. This capability accelerates the identification of potential drug candidates and reduces the time and cost associated with bringing new drugs to market. Furthermore, in genomics, quantum computing can analyze genetic data more efficiently, facilitating personalized medicine and the development of targeted therapies.
Other industries, such as logistics and material science, are also poised for transformation. In logistics, quantum computing can optimize supply chain operations by solving complex optimization problems involving numerous variables. In material science, quantum simulations can lead to the discovery of new materials with desired properties, driving advancements in fields ranging from renewable energy to aerospace.
The widespread adoption of scalable quantum computers across these industries will unlock new levels of efficiency and innovation, reshaping the technological landscape and offering solutions to some of the most pressing challenges of our time.
Real-world examples and case studies
Real-world examples and case studies
Example 1: Quantum Cryptography in Securing Communications
Scalable quantum computers are transforming the field of cryptography by providing new methods for securing communications. One notable example is the implementation of quantum key distribution (QKD) networks. QKD leverages the principles of quantum mechanics to create secure communication channels that are immune to eavesdropping. In China, the deployment of the Beijing-Shanghai QKD network demonstrates the potential of quantum cryptography to secure long-distance communication. This network spans over 2000 kilometers and integrates quantum cryptographic techniques with classical infrastructure, offering a practical solution for safeguarding sensitive information. As scalable quantum computers become more prevalent, the adoption of quantum cryptography will likely increase, providing enhanced security for critical data across various industries.
Example 2: Quantum Algorithms in Financial Modeling
The financial sector is leveraging scalable quantum computers to optimize complex financial models. For instance, Barclays has partnered with IBM to explore the use of quantum algorithms for portfolio optimization. By utilizing quantum algorithms, Barclays aims to improve the accuracy of risk assessments and enhance the performance of investment portfolios. This collaboration highlights the potential of scalable quantum computing to revolutionize financial modeling by providing more precise and efficient solutions. As quantum computing technology advances, financial institutions are expected to increasingly adopt these tools to gain a competitive advantage in the market.
Example 3: Drug Discovery and Genomics
Click here to read our expertly curated top picks!
Overcoming technical obstacles in quantum scalability
Challenges in Implementing Scalable Quantum Computers
Despite significant advancements, implementing scalable quantum computers faces several technical obstacles that must be addressed for widespread adoption. One of the primary challenges is quantum decoherence, a phenomenon where qubits lose their quantum properties due to interactions with their environment. Decoherence limits the time available for quantum computations and affects the accuracy of results. Researchers are actively working on developing techniques to extend coherence times, such as isolating qubits from external noise and using error correction protocols.
Error rates pose another significant challenge in scaling quantum systems. Quantum computations are highly sensitive to errors, and even a single error can lead to incorrect results. Achieving low error rates is crucial for the reliability of quantum computers, especially when performing complex calculations. Researchers are exploring various approaches to reduce error rates, such as improving qubit designs and developing more effective error correction techniques.
The scalability of qubit systems is also a constraint, as increasing the number of qubits while maintaining their coherence is a complex task. Current technologies face limitations in terms of qubit connectivity and control, making it difficult to build large-scale quantum systems. Overcoming these limitations requires innovations in qubit architecture and the development of new materials that support coherent qubit interactions.
Addressing these technical obstacles is essential for realizing the full potential of scalable quantum computers. Continued research and collaboration across disciplines will be crucial in overcoming these challenges and advancing the field of quantum computing.
Potential Solutions and Current Research
Researchers are actively pursuing potential solutions to overcome the challenges of implementing scalable quantum computers. One promising approach is the development of advanced quantum error correction techniques. These techniques involve using additional qubits to detect and correct errors in quantum computations, enhancing the reliability of quantum systems. Recent advancements in error correction codes, such as the surface code, have shown promise in improving fault tolerance and reducing error rates.
Interdisciplinary efforts are also underway to develop new materials and architectures for scalable quantum computing. Researchers are exploring the use of topological qubits, which offer inherent error resistance by storing information in the global properties of the quantum state. Topological qubits have the potential to improve scalability by reducing the need for error correction resources. Additionally, advancements in materials science are focused on developing superconducting materials and novel qubit designs that enhance coherence times and minimize environmental noise.
Current research is also exploring hybrid computing approaches that integrate classical and quantum systems. These approaches leverage the strengths of both paradigms, using classical processors to manage tasks like error correction and state preparation, while quantum processors tackle complex computations. This synergy not only boosts the performance of quantum systems but also provides a practical pathway for integrating quantum computing into existing computational frameworks.
By addressing these challenges and exploring innovative solutions, researchers are paving the way for scalable quantum computers that can revolutionize industries and solve complex problems efficiently. Continued collaboration and investment in quantum research will be essential for driving the development of scalable quantum systems and realizing their transformative potential.
Future directions in scalable quantum computing
Forecasting Developments and Breakthroughs
The future of scalable quantum computing is poised for exciting developments and breakthroughs that will shape the landscape of technology and industry. Over the next decade, advancements in qubit technology, error correction, and quantum algorithms are expected to drive the scalability of quantum systems to new heights. The integration of topological qubits and novel qubit designs will enhance error resistance and coherence times, making large-scale quantum systems more feasible.
Quantum algorithms will continue to evolve, offering exponential speedups for a wider range of applications. As researchers refine existing algorithms and develop new ones, the potential for quantum computing to solve complex problems in cryptography, optimization, and material science will expand. These advancements will open new avenues for quantum computing applications across various industries, transforming how businesses operate and innovate.
The emergence of hybrid computing systems, which seamlessly integrate classical and quantum processors, will further enhance the scalability and practicality of quantum computing. These systems will allow organizations to leverage the strengths of both paradigms, optimizing performance and resource utilization. As hybrid systems become more widespread, the adoption of quantum computing is expected to accelerate, driving innovation and efficiency across sectors.
Overall, the next decade promises to be a transformative period for scalable quantum computing, with breakthroughs that will unlock new possibilities and reshape the technological landscape. Continued investment in research and collaboration across disciplines will be crucial for realizing the full potential of quantum computing and driving its integration into real-world applications.
Implications of Scalable Quantum Computing
The implications of scalable quantum computing are far-reaching, with the potential to impact society, technology, and the global economy in profound ways. As scalable quantum systems become more prevalent, industries will experience a paradigm shift in how complex problems are approached and solved. Sectors such as finance, healthcare, and logistics will benefit from enhanced computational capabilities, leading to improved efficiency, cost savings, and innovation.
On a societal level, scalable quantum computing has the potential to drive advancements in scientific research and discovery. Quantum simulations of molecular interactions, for example, could lead to breakthroughs in drug discovery and material science, addressing some of the world's most pressing challenges. Additionally, the ability to process vast amounts of data and perform complex calculations will enable new insights and solutions in fields such as climate modeling and renewable energy.
From an economic perspective, countries that lead in quantum computing research and development will gain a strategic advantage in the global market. The ability to harness the power of scalable quantum computing will drive competitiveness and economic growth, positioning these countries as leaders in innovation and technology. As quantum computing continues to evolve, the implications for global trade, security, and collaboration will become increasingly significant.
Overall, scalable quantum computing holds the potential to redefine industries and drive advancements across multiple domains, offering solutions to complex challenges and unlocking new opportunities for innovation and growth.
Click here to read our expertly curated top picks!
Ethical and societal impact of scalable quantum computers
Broader Consequences of Quantum Computing Integration
The integration of scalable quantum computers into society brings with it a host of ethical and societal considerations that must be addressed to ensure responsible and equitable adoption. One of the primary ethical concerns is the potential for quantum computing to disrupt existing encryption methods, posing significant risks to data privacy and security. As quantum computers become more capable of breaking traditional encryption schemes, there is an urgent need to develop new cryptographic protocols that can withstand quantum attacks. This requires collaboration between researchers, policymakers, and industry stakeholders to ensure data protection and privacy in a quantum-enabled world.
The socio-economic impacts of quantum computing integration are also significant. While quantum computing has the potential to drive innovation and economic growth, it may also lead to job displacement as automation and advanced algorithms replace certain roles. To mitigate these impacts, it is crucial to invest in education and workforce development programs that equip individuals with the skills needed to thrive in a quantum-driven economy. Additionally, efforts must be made to ensure that the benefits of quantum computing are distributed equitably, addressing the digital divide and preventing disparities in access to quantum technologies.
Another ethical consideration is the potential for quantum computing to exacerbate surveillance and control. The ability to process vast amounts of data and perform complex calculations could enable more sophisticated surveillance systems, raising concerns about privacy and civil liberties. It is essential to establish governance frameworks that balance the benefits of quantum computing with protections for individual rights and freedoms. By addressing these ethical and societal considerations, the integration of scalable quantum computers can be guided by principles of responsibility, equity, and inclusivity.
Privacy, Security, and Governance Issues
The deployment of scalable quantum computers raises significant privacy, security, and governance issues that require careful consideration and proactive measures. One of the primary challenges is the potential for quantum computers to break existing encryption schemes, posing risks to data privacy and security. As quantum technology advances, it is crucial to develop quantum-resistant cryptographic protocols that can protect sensitive information from quantum attacks. This requires collaboration between researchers, policymakers, and industry stakeholders to ensure the development and implementation of robust security measures.
Governance frameworks must also be established to address the ethical and societal implications of quantum computing. These frameworks should include guidelines for the responsible use of quantum technology, balancing the benefits of innovation with protections for individual rights and freedoms. Policymakers must work closely with industry leaders and researchers to develop regulations that prevent misuse and ensure equitable access to quantum technologies.
In addition to privacy and security concerns, the integration of quantum computing poses challenges related to intellectual property and data ownership. As quantum technology becomes more prevalent, it is essential to establish clear guidelines for the ownership and use of quantum-related data and intellectual property. This requires international collaboration and the development of standardized practices to ensure fair and transparent handling of quantum data.
By addressing these privacy, security, and governance issues, the deployment of scalable quantum computers can be guided by principles of responsibility, transparency, and accountability. This will help ensure that the benefits of quantum computing are realized while safeguarding individual rights and societal interests.
Click here to read our expertly curated top picks!
Tips: do's and don'ts in engaging with quantum computing
Do's | Don'ts |
---|---|
Stay updated with the latest research | Ignore the ethical implications |
Collaborate across disciplines | Underestimate complexity |
Focus on practical applications | Prioritize theory over practice |
Explore diverse quantum technologies | Limit learning to one approach |
Click here to read our expertly curated top picks!
Summarizing insights on scalable quantum computers
Recapping the Transformative Potential of Quantum Scalability
Throughout this exploration, we have highlighted the transformative potential of scalable quantum computing and its ability to revolutionize industries by solving complex problems more efficiently than classical systems. Scalable quantum computers leverage the principles of quantum mechanics, such as superposition and entanglement, to perform computations at unprecedented speeds. Achieving scalability is crucial for realizing the full potential of quantum computing, enabling real-world applications and breakthroughs across various sectors.
The implications of scalable quantum computing are far-reaching, with the potential to impact society, technology, and the global economy in profound ways. From enhancing encryption methods and optimizing financial models to accelerating drug discovery and revolutionizing supply chain operations, scalable quantum computers offer solutions to complex challenges and unlock new opportunities for innovation and growth.
As the field of quantum computing continues to evolve, ongoing research and collaboration will be essential for overcoming technical challenges and driving the development of scalable quantum systems. By addressing these challenges and exploring innovative solutions, scalable quantum computing can pave the way for a new era of technological advancement, reshaping the technological landscape and offering solutions to some of the most pressing challenges of our time.
All You Need for Project Management is in Meegle
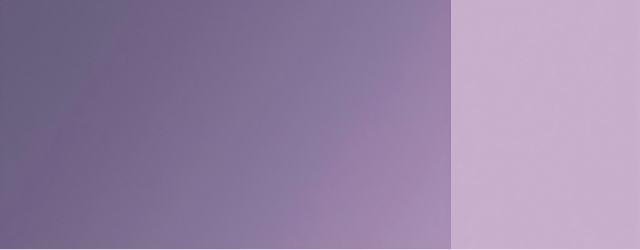
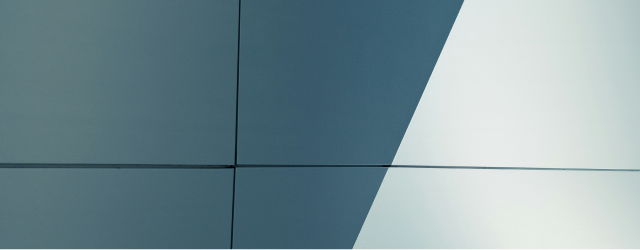
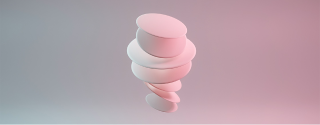
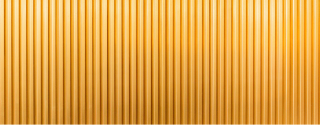
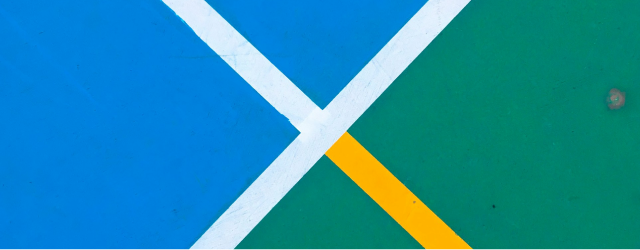
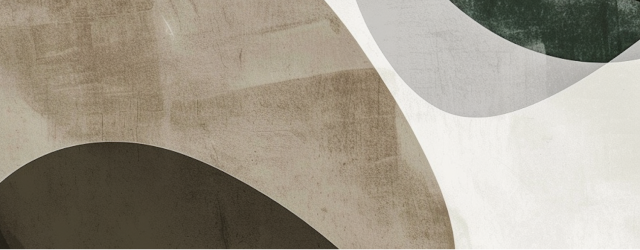
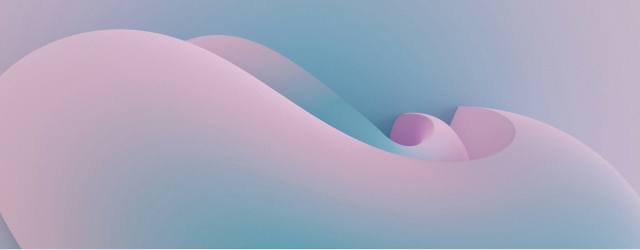
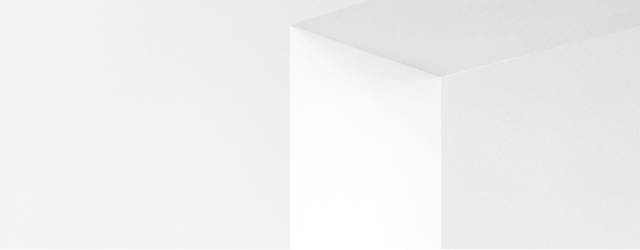