Aerospace Engineering
Enhance industry performance and operational intelligence with Aerospace Engineering, a key component in the realm of Digital Twin technology.
A Foundation of Innovation and Exploration
Aerospace Engineering is a specialized branch of engineering that focuses on the development and maintenance of aircraft and spacecraft. It encompasses two main disciplines: aeronautical engineering, which pertains to vehicles operating within the Earth's atmosphere, and astronautical engineering, which deals with vehicles operating beyond the Earth's atmosphere. The field is underpinned by fundamental principles of physics and mathematics, with a strong emphasis on fluid dynamics, materials science, structural analysis, propulsion, and avionics. These principles are crucial in designing and testing aircraft and spacecraft that are both reliable and efficient.
The role of Aerospace Engineering in technological advancements cannot be understated. Its contributions span from the development of cutting-edge aviation technology to the design of spacecraft that explore the far reaches of our solar system. Aerospace engineers apply their expertise to improve flight safety, fuel efficiency, and environmental impact, driving innovation in both military and civilian aviation sectors. The exploration of space has also been significantly advanced through aerospace engineering, resulting in technological spinoffs that benefit various industries, including telecommunications, weather forecasting, and even consumer electronics.
At the heart of this field's transformative power is Digital Twin Technology, a sophisticated digital model that accurately replicates physical assets, processes, or systems. In Aerospace Engineering, digital twins are utilized to simulate aircraft behavior under different conditions, predict maintenance needs, and optimize design processes. By integrating Digital Twin Technology, Aerospace Engineering is poised to further revolutionize manufacturing processes, enhance aircraft performance, and reduce operational costs. This integration represents a paradigm shift where virtual testing and predictive analytics become integral to the engineering lifecycle, offering unprecedented insights and efficiencies.
Experience a New Level of Productivity in Meegle
Concept and definition
Detailed Definition of Aerospace Engineering
At its core, Aerospace Engineering is the discipline dealing with the design, development, and production of air and spacecraft. This field involves numerous sub-disciplines, including aerodynamics, propulsion, avionics, materials science, structural analysis, and manufacturing. Each of these areas plays a crucial role in ensuring that aerospace vehicles are capable of withstanding the unique challenges of flight and space exploration. Aerospace Engineering is characterized by its rigorous application of scientific principles to solve complex engineering problems, whether it’s designing a new type of wing for enhanced lift or developing a propulsion system for reaching Mars.
The scope of Aerospace Engineering is vast, covering everything from the design and testing of individual components to the integration of these components into a fully functioning system. Aerodynamics, for example, involves the study of airflow over an aircraft's surfaces to minimize drag and maximize lift. Propulsion focuses on developing engines that provide thrust, whether through jet propulsion for aircraft or rocket propulsion for spacecraft. Avionics encompasses the electronic systems used for navigation, communication, and control. Structural analysis ensures that the aircraft or spacecraft can withstand the stresses encountered during flight, while materials science focuses on developing lightweight yet strong materials.
Integration with the Digital Twin Framework
Digital Twin Technology represents a significant advancement in how Aerospace Engineering is conducted. A digital twin is a dynamic, virtual representation of a physical object or system, capturing its attributes, behaviors, and interactions. This digital model can be used to monitor performance, predict outcomes, and optimize processes by analyzing real-time data and historical trends. In aerospace, digital twins are employed to simulate and predict the behavior of aircraft and spacecraft, enabling engineers to foresee potential issues and optimize design and operation.
The synergy between Aerospace Engineering and Digital Twin Technology is profound. By combining physical engineering principles with advanced digital simulations, engineers can create more accurate models of how an aircraft or spacecraft will perform in the field. This integration allows for enhanced predictive maintenance capabilities, where potential issues can be identified and addressed before they result in downtime or accidents. Furthermore, digital twins enable more efficient design processes by allowing engineers to test and refine designs virtually before physical prototypes are constructed.
In industrial applications, this synergy leads to significant improvements in efficiency and cost-effectiveness. For example, the use of digital twins in aircraft maintenance can reduce downtime by predicting and preventing mechanical failures. In manufacturing, digital twins can optimize production processes, resulting in higher quality products and reduced waste. The integration of Aerospace Engineering and Digital Twin Technology not only enhances the capabilities of individual systems but also transforms entire industries by enabling smarter, more data-driven decision-making.
Historical development
Evolution in Aerospace Engineering
The evolution of Aerospace Engineering traces a fascinating journey from the early days of flight to the advanced technologies of today. Key historical milestones in this field highlight the relentless pursuit of innovation and the drive to explore beyond our planet. The Wright brothers' first powered flight in 1903 marked the dawn of modern aviation, setting the stage for rapid advancements in aircraft design and aerodynamics. The development of the jet engine in the mid-20th century revolutionized air travel, enabling faster and more efficient transportation.
The space race of the 1960s further accelerated the evolution of Aerospace Engineering. The successful launch of Sputnik by the Soviet Union in 1957, followed by the Apollo missions that culminated in the moon landing in 1969, demonstrated the potential of aerospace technology to reach beyond Earth's atmosphere. These achievements spurred further advancements in rocket propulsion, materials science, and systems engineering. In subsequent decades, the development of reusable spacecraft, such as the Space Shuttle, showcased the potential for cost-effective space exploration.
The evolution of engineering practices and methodologies within Aerospace Engineering has been characterized by an increasing emphasis on simulation and modeling. The advent of computer-aided design (CAD) and computational fluid dynamics (CFD) has enabled engineers to test and refine designs virtually, reducing the need for costly physical prototypes. Today, Aerospace Engineering continues to push the boundaries of what is possible, with ongoing research into supersonic and hypersonic flight, autonomous aircraft, and sustainable aviation technologies.
Milestones in Digital Twin Technology
The concept of Digital Twin Technology has roots in the early days of simulation and modeling, but it has only recently gained prominence as a transformative tool for various industries. One of the earliest examples of digital twin usage was NASA's Apollo program, where virtual replicas of spacecraft were used to simulate and troubleshoot potential issues. This early application laid the groundwork for the digital twin concept by demonstrating the value of accurate virtual models in enhancing system performance and reliability.
In the decades following the Apollo program, advancements in computing power and data analytics paved the way for the development of more sophisticated digital twins. The rise of the Internet of Things (IoT) and big data analytics in the 21st century has further propelled the adoption of digital twin technology. Today, digital twins are used across various industries, from aerospace and automotive to healthcare and manufacturing, to improve operational efficiency and reduce costs.
The influence of digital twin milestones on Aerospace Engineering is significant. By providing a virtual representation of an aircraft or spacecraft, digital twins enable engineers to simulate and analyze real-world conditions, predict maintenance needs, and optimize design processes. This capability has transformed the way aerospace systems are developed, tested, and operated, leading to enhanced safety, performance, and cost-effectiveness. As digital twin technology continues to evolve, it promises to further revolutionize Aerospace Engineering and its applications in various industries.
Core applications
Applications in aerospace and other industries
Applications in aerospace and other industries
The applications of Aerospace Engineering are not confined to the skies and beyond; they have permeated various industries, showcasing the versatility and impact of aerospace principles. In aviation, aerospace engineering is instrumental in designing and manufacturing commercial and military aircraft, ensuring safety, efficiency, and sustainability. The defense sector benefits from advanced aerospace technologies in the development of unmanned aerial vehicles (UAVs), missiles, and sophisticated avionics systems that enhance situational awareness and operational capabilities.
In space exploration, aerospace engineering underpins the development of satellites, space probes, and manned spacecraft. This field's contributions enable critical missions, from placing communication satellites in orbit to sending rovers to Mars. The knowledge derived from these endeavors often translates into technological advancements that benefit other sectors. For instance, the miniaturization of components for space missions has spurred innovations in consumer electronics and telecommunications.
Beyond traditional aerospace applications, the principles of aerospace engineering have found utility in cross-industry applications. In the automotive sector, aerospace techniques are employed to improve vehicle aerodynamics, resulting in enhanced fuel efficiency and performance. The manufacturing industry leverages aerospace methodologies to develop high-precision machinery and equipment. Even the healthcare sector benefits from aerospace engineering, where principles of fluid dynamics and materials science contribute to the development of advanced medical devices, such as prosthetics and imaging equipment.
Business impact and real-world usage
Business impact and real-world usage
The real-world impact of integrating Digital Twin Technology with Aerospace Engineering is profound, offering quantifiable business outcomes across various sectors. The aviation industry, for instance, has seen significant improvements in maintenance operations through digital twins. By creating virtual replicas of aircraft, airlines can predict when components might fail and schedule maintenance proactively, reducing downtime and operational costs. This predictive maintenance approach not only increases aircraft availability but also enhances safety by preventing unexpected failures.
In manufacturing, digital twins facilitate the optimization of production processes, leading to improved product quality and reduced waste. For example, a manufacturing plant can use digital twins to simulate production line changes and assess their impact before implementation, minimizing disruptions and maximizing efficiency. This capability is particularly valuable in industries where precision and reliability are paramount, such as aerospace and automotive manufacturing.
The business impact extends to the healthcare industry, where digital twins are used to model the human body and simulate medical procedures. This application enables healthcare professionals to plan surgeries with greater accuracy, improving patient outcomes. Moreover, digital twins can aid in the development of personalized medicine by simulating how different treatments might affect individual patients, leading to more effective and tailored healthcare solutions.
Click here to read our expertly curated top picks!
Benefits and advantages
Operational Benefits of Aerospace Engineering
The operational benefits of Aerospace Engineering are numerous, offering significant advantages in terms of efficiency, safety, and cost-effectiveness. One of the primary benefits is the increased efficiency of aircraft and spacecraft design and operation. Aerospace engineers utilize advanced simulation and modeling techniques to optimize aerodynamic performance, reducing fuel consumption and emissions. This focus on efficiency extends to the manufacturing process, where aerospace principles help streamline production, resulting in faster turnaround times and lower costs.
Safety is another critical area where aerospace engineering excels. The rigorous testing and validation processes employed in this field ensure that aircraft and spacecraft meet the highest safety standards. By simulating various flight conditions and potential failure scenarios, engineers can identify and mitigate risks before they manifest in real-world operations. This proactive approach to safety enhances the reliability of aerospace systems and protects lives, both in the air and in space.
Predictive maintenance is a key advantage enabled by the integration of digital twin technology in aerospace engineering. By continuously monitoring the condition of aircraft and spacecraft, digital twins can anticipate maintenance needs and schedule repairs before issues arise. This capability not only reduces downtime and operational disruptions but also extends the lifespan of aerospace assets, maximizing their value and return on investment. Additionally, the use of digital twins in design and testing processes leads to better performance optimization, ensuring that aerospace systems operate at peak efficiency throughout their lifecycle.
Comparative Analysis: Pre and Post Implementation
The transformative impact of integrating Aerospace Engineering with digital twin technology can be clearly observed through comparative analysis of pre and post-implementation scenarios. Before the adoption of digital twins, aerospace systems relied heavily on physical prototypes and testing, which were both time-consuming and costly. The absence of predictive analytics meant that maintenance was often reactive, resulting in unexpected downtime and higher operational costs.
Post-implementation, the benefits of digital twins are evident in the enhanced efficiency and effectiveness of aerospace operations. For instance, the use of digital twins in aircraft design allows engineers to conduct virtual testing, significantly reducing the need for physical prototypes and accelerating the design process. This virtual approach not only saves time and resources but also enables more innovative designs, as engineers can quickly iterate and test various concepts.
In terms of maintenance, the shift from reactive to predictive maintenance has led to substantial cost savings and increased aircraft availability. Airlines that have implemented digital twin technology report fewer unscheduled maintenance events and lower maintenance costs, as issues are identified and addressed before they lead to failures. This proactive approach also enhances safety, as potential risks are mitigated before they pose a threat to operations.
The post-implementation era is characterized by improved productivity and innovation, driven by the insights provided by digital twins. These virtual models enable aerospace engineers to optimize performance, reduce environmental impact, and develop more sustainable technologies. The comparative analysis clearly demonstrates the value of integrating aerospace engineering with digital twin technology, highlighting the potential for transformative improvements in efficiency, safety, and cost-effectiveness across the industry.
Integration strategies
Effective Integration with Existing Systems
Successfully integrating Aerospace Engineering with existing systems requires a strategic approach that considers the unique challenges and opportunities of each organization. One of the key strategies is to adopt a phased implementation process, where digital twin technology is gradually introduced across different aspects of aerospace operations. This approach allows organizations to manage change effectively, minimizing disruptions and ensuring a smooth transition to new methodologies.
To facilitate seamless integration, it is essential to invest in the right tools and technologies. Digital twin platforms that offer robust data analytics capabilities and real-time monitoring are crucial for capturing and analyzing the vast amounts of data generated by aerospace systems. Additionally, interoperability with existing systems is paramount, as digital twins must be able to communicate and exchange data with legacy systems to provide accurate and actionable insights.
Collaboration with industry experts and stakeholders is another critical factor in successful integration. By engaging with experts in digital twin technology and aerospace engineering, organizations can gain valuable insights and guidance on best practices and potential pitfalls. This collaboration extends to partnerships with technology providers, who can offer tailored solutions and support throughout the integration process.
Challenges and Considerations
Despite the numerous benefits, adopting Aerospace Engineering and digital twin technology presents certain challenges that organizations must address. One of the primary challenges is the complexity of integrating digital twins with existing systems, particularly in organizations with legacy infrastructure. Ensuring compatibility and interoperability between new and existing systems can be a daunting task, requiring significant investment in time and resources.
Data security and privacy are also critical considerations, as digital twins rely on real-time data collection and analysis. Organizations must implement robust security measures to protect sensitive data from cyber threats and ensure compliance with industry regulations. This may involve investing in advanced cybersecurity technologies and developing comprehensive data governance policies.
Another challenge is the need for skilled professionals who can effectively manage and leverage digital twin technology. The integration of digital twins requires expertise in both aerospace engineering and data analytics, necessitating ongoing training and development for existing staff or the recruitment of new talent. Organizations must prioritize workforce development to ensure that they have the necessary skills and knowledge to maximize the benefits of digital twin technology.
To overcome these challenges, organizations can adopt best practices such as conducting thorough research and planning before implementation, investing in training and development, and fostering a culture of innovation and continuous improvement. By addressing these considerations, organizations can successfully navigate the complexities of integrating aerospace engineering with digital twin technology and unlock its full potential.
Future trends and predictions
Emerging Developments in Aerospace Engineering
The future of Aerospace Engineering is poised to be shaped by a host of emerging developments and innovations that promise to redefine the industry. One of the most significant trends is the increasing role of artificial intelligence (AI) and machine learning in aerospace design and operations. These technologies are being leveraged to enhance predictive maintenance, optimize flight paths, and improve decision-making processes. AI-driven algorithms can analyze vast amounts of data in real-time, providing actionable insights that enhance efficiency, safety, and performance.
Another exciting development is the exploration of sustainable aviation technologies, driven by the global push for environmental conservation. Aerospace engineers are exploring alternative fuels, such as biofuels and hydrogen, as well as electric propulsion systems, to reduce the carbon footprint of air travel. The development of lightweight materials and aerodynamic designs also contributes to greater fuel efficiency and reduced emissions. These innovations align with the industry’s commitment to achieving carbon-neutral growth and eventually net-zero emissions.
The advent of advanced manufacturing techniques, such as additive manufacturing (3D printing), is transforming the production of aerospace components. This technology allows for the creation of complex, lightweight structures that are both cost-effective and efficient. Additive manufacturing also enables rapid prototyping and customization, accelerating the design and development process.
Future Impacts and Industry Applications
The future impacts of Aerospace Engineering and digital twin technology extend far beyond the traditional aerospace sector, with potential applications across various industries. In the automotive industry, the principles of aerospace engineering are being applied to develop more efficient and aerodynamic vehicles. Digital twins can simulate vehicle performance under different conditions, optimizing design and enhancing safety features. As the industry moves towards autonomous and electric vehicles, the integration of aerospace methodologies will be crucial in addressing the challenges of energy efficiency and system reliability.
In the healthcare sector, the application of aerospace engineering principles in developing advanced medical devices and equipment is expected to continue. Digital twins offer the potential to revolutionize patient care by creating virtual models of the human body, enabling personalized treatment plans and improving surgical outcomes. This technology can also aid in the development of prosthetics and implants, enhancing functionality and patient comfort.
The construction industry stands to benefit from aerospace engineering and digital twin technology through the development of smart buildings and infrastructure. By applying aerospace methodologies, engineers can design structures that are more resilient to environmental challenges while optimizing energy efficiency. Digital twins enable real-time monitoring and management of building systems, enhancing safety, comfort, and sustainability.
In conclusion, the future trends and predictions for aerospace engineering and digital twin technology highlight their transformative potential across diverse industries. As these technologies continue to evolve, they promise to drive innovation, enhance efficiency, and address some of the most pressing challenges facing our world today.
Use cases and case studies
Case Study: Aerospace Engineering in Aviation
The application of Digital Twin Technology in the aviation industry has been transformative, particularly in the realm of aircraft design and maintenance. A notable example is the use of digital twins by Boeing in the development of their 787 Dreamliner. By creating a digital twin of the aircraft, Boeing was able to simulate and test various design iterations, optimizing performance and reducing the need for physical prototypes. This approach not only accelerated the design process but also resulted in a more efficient and environmentally friendly aircraft.
In terms of maintenance, digital twins have enabled airlines to adopt predictive maintenance strategies, significantly reducing downtime and operational costs. For instance, GE Aviation uses digital twins to monitor the health of aircraft engines, predicting failures before they occur and scheduling maintenance proactively. This capability has led to increased aircraft availability and enhanced safety, as potential issues are identified and addressed before they impact operations.
Case Study: Automotive Industry
The Automotive Industry has also benefited from the integration of aerospace engineering principles and digital twin technology. A prime example is the development of electric vehicles (EVs) by companies like Tesla. Aerospace methodologies have been applied to improve the aerodynamics and efficiency of EVs, resulting in extended range and performance. Digital twins are used to simulate vehicle behavior under different conditions, optimizing design and enhancing safety features.
In manufacturing, digital twins enable automotive companies to simulate production processes, identify bottlenecks, and optimize operations. This capability has led to improved product quality and reduced waste, resulting in significant cost savings. Additionally, the use of digital twins in the testing and validation of autonomous vehicles has accelerated the development of self-driving technology, bringing the industry closer to widespread adoption.
Case Study: Healthcare Innovations
In the Healthcare Industry, the application of aerospace engineering principles and digital twin technology has led to significant advancements in the development of medical devices and treatment plans. One such example is the use of digital twins in orthopedic surgery. By creating a virtual model of a patient's anatomy, surgeons can plan and simulate procedures with greater accuracy, improving surgical outcomes and reducing recovery times.
Digital twins are also being used to develop personalized treatment plans, particularly in the field of oncology. By modeling how different treatments might affect an individual patient, healthcare providers can tailor therapies to achieve the best possible outcomes. This personalized approach is expected to revolutionize patient care, leading to more effective and efficient healthcare solutions.
Do's and don'ts
Do's | Don'ts |
---|---|
Conduct thorough research before implementation | Rush into adoption without a clear strategy |
Invest in training and development | Ignore the need for skilled professionals |
Collaborate with industry experts | Overlook integration challenges with existing systems |
Conclusion
In conclusion, the integration of Aerospace Engineering with digital twin technology presents a transformative potential that extends beyond traditional aerospace applications. This powerful synergy offers significant benefits in terms of efficiency, safety, and cost-effectiveness, promising to revolutionize diverse industries from aviation and automotive to healthcare and manufacturing. As organizations embrace this innovative approach, they unlock new opportunities for predictive maintenance, performance optimization, and enhanced design processes. The success of this integration relies on strategic planning, investment in technology and training, and a commitment to continuous improvement. By harnessing the full potential of aerospace engineering and digital twin technology, industries can achieve unprecedented levels of industrial efficiency, paving the way for a more sustainable and innovative future.
Faqs
What is Aerospace Engineering?
Aerospace Engineering is a specialized field of engineering that focuses on the design, development, and production of aircraft and spacecraft. It encompasses two main disciplines: aeronautical engineering, which deals with vehicles operating within the Earth's atmosphere, and astronautical engineering, which pertains to vehicles operating beyond the Earth's atmosphere. Aerospace engineers apply principles of physics and mathematics to solve complex engineering challenges and advance aviation and space exploration technologies.
How does Aerospace Engineering integrate with Digital Twin Technology?
The integration of Aerospace Engineering with Digital Twin Technology involves creating virtual replicas of physical aerospace systems, such as aircraft and spacecraft. These digital twins enable engineers to simulate, monitor, and analyze system performance in real-time, providing valuable insights for predictive maintenance, design optimization, and operational efficiency. By combining physical engineering principles with advanced digital simulations, this integration enhances safety, performance, and cost-effectiveness across aerospace operations.
What are the key benefits of implementing these technologies?
Implementing Aerospace Engineering and digital twin technology offers several key benefits, including increased efficiency, safety, and cost-effectiveness. By leveraging digital twins, organizations can predict maintenance needs, optimize design processes, and reduce downtime, leading to enhanced performance and reliability. This proactive approach also improves safety by identifying and mitigating potential risks before they impact operations. Additionally, the integration of these technologies enables more sustainable and innovative solutions across various industries.
What industries can benefit from Aerospace Engineering?
Aerospace Engineering benefits a wide range of industries beyond traditional aviation and space exploration. In the automotive industry, aerospace principles are applied to improve vehicle aerodynamics and efficiency. The manufacturing sector utilizes aerospace methodologies for high-precision production processes. In healthcare, aerospace engineering contributes to the development of advanced medical devices and personalized treatment plans. Other industries, such as defense and telecommunications, also leverage aerospace technologies for enhanced capabilities and performance.
What are the challenges in adopting Aerospace Engineering?
Adopting Aerospace Engineering and digital twin technology presents challenges such as integrating new systems with existing infrastructure, ensuring data security and privacy, and addressing the need for skilled professionals. Organizations must invest in upgrading legacy systems, implementing robust cybersecurity measures, and providing training and development programs to equip staff with the necessary skills. By addressing these challenges through strategic planning and collaboration, organizations can successfully navigate the complexities of adoption and unlock the full potential of these technologies.
Experience a New Level of Productivity in Meegle
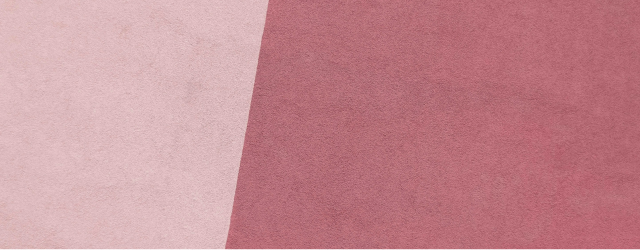
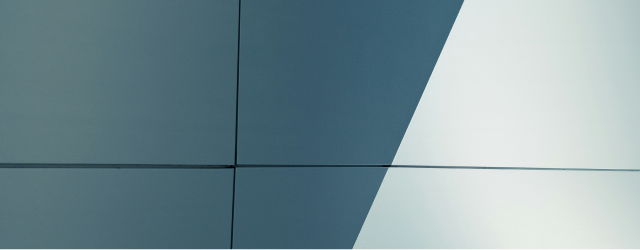
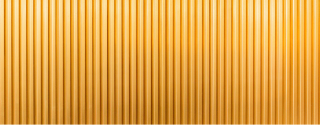
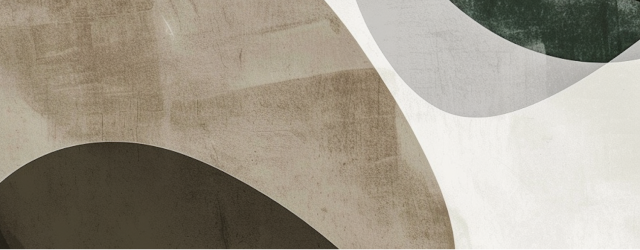
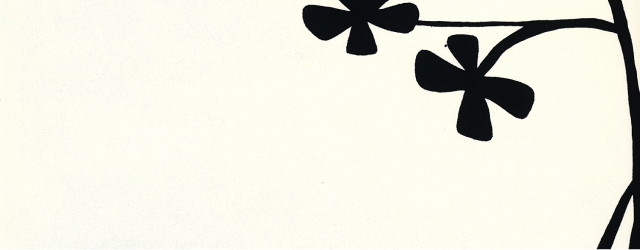
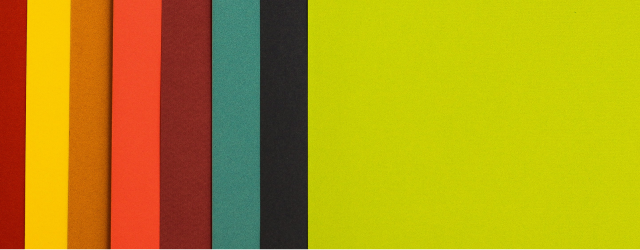
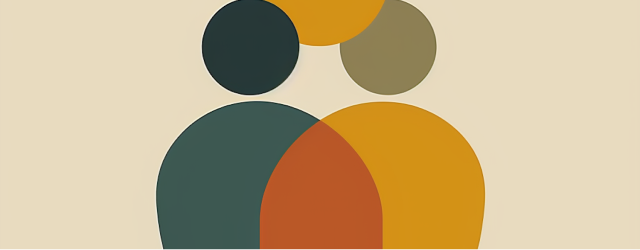